Abstract—In the past 40 years, alpine skiing equipment has improved significantly in its ability to help prevent injuries to skiers. However, Anterior Cruciate Ligament (ACL) injuries have drastically increased during this time period. Manufacturers design skis, boots, and especially bindings to prevent severe injuries, but the equipment needs to do a better job at protecting our knees. This paper will discuss the development of ski equipment—bindings in particular—with the goal of reducing ACL injuries. These injuries typically occur because of the application of a critical load to the knee during a fall. Since binding design primarily stems from the push to prevent tibia fractures in skiers during the 1970's, current bindings on the market do not account for ACL injuries. Therefore, to reduce ACL injuries, many researchers have discussed the possibility of improving binding function by implementing additional degrees of freedom to the current industry standard of two [11]. By implementing designs that take into account oblique loads, more advanced bindings may significantly decrease the risk of a severe knee sprain. In our paper about the improvement of ski bindings to reduce knee injuries, we will examine current bindings and why they are inadequate at preventing knee injuries. In a connected vein of research, we will examine specific mechanisms associated with ACL injuries in skiing to help explain why certain binding improvements may be effective. Finally, we will present the potential improvements in detail and attempt to analyze their effectiveness, concentrating particularly on the aforementioned "degrees of freedom" development and more comprehensive load sensing.
STRIVING FOR A SAFER SKI BINDING
One could argue that sports are the most popular hobby in American culture. Viewers revere the athletic talent on display every day of the week. Some of the biggest events on earth such as the World Cup and the Olympics foster friendly competition and international cooperation. On a smaller scale, sports have a number of benefits for individuals. People who actively participate in sports benefit from a number of perks including decreased chance of chronic disease, longer average life span, and overall better mental health [2]. However, many sporting activities also come with the inherent risk of injury. Football players risk brain damage. Tennis players risk the painful ligament damage known as tennis elbow. Skiers in particular risk ACL injuries among many others. In all these cases, and especially skiing, technological development can go a long way in the reduction of injuries. Skiing performance and safety depends highly on technology, and an improvement of the technology can have a powerful effect on the safety of participants.
Although skiing equipment has drastically reduced the number of overall injuries over the past 40 years, specific injuries have actually become much more prevalent. Of these, the most common is the severe knee sprain, usually of the anterior crucial ligament (ACL). Since 1972, severe ligament sprains in the knee have increased by over 200% during alpine skiing [3]. This surge in knee injuries can be understood by looking at the history of skiing leg injuries in general, and how engineers have developed equipment to counteract them.
HISTORY OF ACL INJURIES IN SKIING
Since the advent of the modern "safety" ski binding that reduces lower leg fractures and ankle sprains, ACL tears have become the most prevalent of all injuries in skiing. According to Finch and Kelsall, "the knee is the most common site for alpine skiing injuries, accounting for between 20% and 32% of all injuries" [4]. This is a major problem because an ACL tear is rarely a minor injury; it can potentially limit someone who has suffered the injury for the rest of his or her life. The rehabilitation period for an ACL injury is exceptionally long and arduous compared with most other injuries [5]. For many, especially athletes and manual workers, who depend on their bodies for income, the immense timetable and lasting effects of the injury could be devastating.
One example of a high profile, devastating ACL tear is the case of U.S. skier and once Sochi Olympic hopeful Lindsey Vonn. Early last year, she suffered an ACL tear that doctors told her would not prevent her from competing in the Olympics. However, despite intensive rehabilitation and physical therapy, Vonn's knee was not healthy enough by the time of the Olympic qualifiers, and she had to drop out [6]. Injuries like this can be career-ending for athletes like Vonn. They can also keep those in professions involving construction, delivery, etc, from being able work effectively, which could financially endanger them and their families.
THE ORIGIN OF SKI BINDING DESIGN
The first prototypes of self-releasing ski bindings were designed in the late 1930's in an attempt to reduce tibia fractures and ankle sprains in skiers. Before this time, skiers' boots were attached directly to their skis without any way of breaking free in the event of a crash. Without any kind of release, a skier's leg would be forced into the boot. With large enough force, or sustained force, the skier's tibia would fracture under the pressure. There was a call for bindings that could prevent these injuries. The bindings that emerged in this time period were designed to allow for the boot to release from the ski, minimizing pressure on the skier's leg in the event of a crash, and thus reducing the chance of a lower leg fracture. At first, they consisted of only a release in the toe, but by the 1950's, bindings with additional release mechanisms in the heel began to emerge onto the market. In the late 1960's the modern design of the ski bindings arrived with a step-in heel and pivoting toe. [7]
CONVENTIONAL BINDINGS
Current ski bindings that can be found on almost all of today's skis consist of two main parts: a toepiece and a heelpiece. These are screwed directly into the top of the ski or onto a riser plate which is mounted to the ski. The skier's boot can then be attached to the ski through use of the binding. The binding can be manually released by the skier and is also designed to release during a crash to prevent injury. If a certain pre-set force tolerance due to the twisting motion of the skier's boot in the binding is reached, the binding will release. Most current bindings release in two direction: horizontally at the toe and vertically at the heel as shown in Figure 1 [8]. The release mechanism works using a spring system in both the toepiece and heelpiece. In the toepiece, the spring controls the tightness of the wings (clamps on either side of the toe of the boot that hold it in place). A higher tension setting results in more force necessary to release the binding. Likewise, the heelpiece spring controls how easily the back of the boot can swing upwards out of the binding when a large force is applied to the rear (which is also shown in Figure 2).
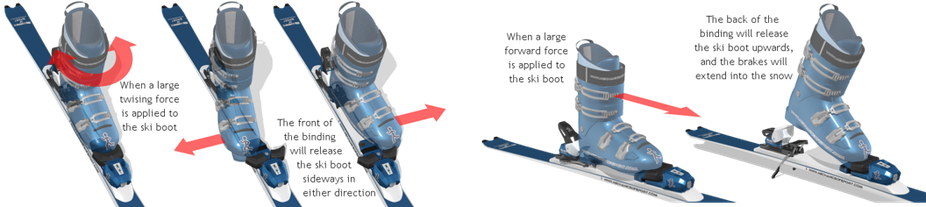
FIGURE 1 [8]. The two degrees of release freedom in conventional ski bindings
There are some binding designs, such as the Rossignol Axial, Axial2, and FKS140 (and 120), which incorporate an additional release degree, a vertical release in the toe as shown in Figure 2 [9]. The vertical release allows the toe to release directly upwards in response to an upward force on the bottom of the ski. But these are not very common because they have been associated with pre-release of the binding when release is not warranted in non-crash situations [9]. For aggressive, advanced-level skiers, this could be the cause of severe injury.
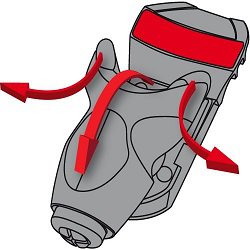
FIGURE 2 [9]. The additional vertical release degree of freedom found in some binding designs
The release tolerances, as well as the build tolerances and other specifications all bindings must meet are defined by Deutsches Institut für Normung (DIN), which is the industry standard for the shape of the binding-boot interface and the release mechanism [9]. Nearly every aspect of binding and boot designs are addressed by DIN. The most important thing DIN provides is a common tension scale for the tightness of bindings' retention settings, which range from zero to 18 [8]. The higher the DIN value, the tighter the binding spring tension. This scale allows skiers to switch to different models or brands of bindings without worrying about finding new settings or being concerned about unwanted injury that could occur as the result of improperly set bindings. All bindings on the market have tension adjustments that are standardized to the scale specified by DIN, and there are many charts, calculators, and tables available online and in ski shops that help skiers find their individual recommended DIN settings. A skier's recommended DIN setting is based on the combined factors of his or her weight, height, ability level, age, and boot sole length (BSL), which is the length from the tip of the toe of the boot to the very rear of the heel [10]. Although the system set up by DIN is satisfactory, there are still problems with current bindings which can lead to injury even when the bindings are set correctly to the recommended DIN settings.
Problems with Current Bindings
Current ski bindings do succeed in minimizing the lower leg injuries they were designed to address. Over the decades that skiing became popular and binding designs have improved, tibia fractures and ankle injuries have decreased significantly as shown in Figure 3, a graph from the Journal of ASTM International [11]. However, the amount of knee injuries suffered by skiers each year has, actually increased which is unexpected considering many years of product design evolution [11]. This is mainly due to the technical purpose of ski bindings. The original safe ski bindings were designed only to reduce lower leg fractures; no other injuries were addressed at that time. Unfortunately, not much has changed in binding design since then; bindings still consist of a separate heelpiece and toepiece, each with just one degree of freedom of release, neither of which address knee injuries.
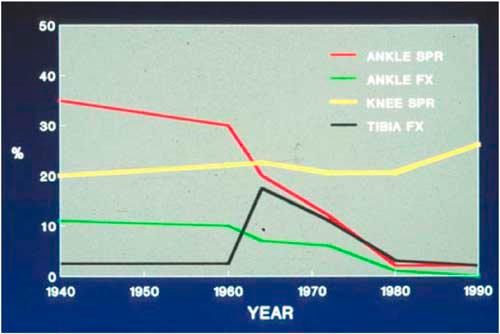
FIGURE 3 [11]. Graph of different leg injury frequencies in skiing from 1940-1990 (knee sprain frequency is the yellow line)
Contrary to popular belief, ski bindings do very little to prevent ACL injuries because of the sheer mechanics of the injury [5]. ACL injury mechanics are very different from lower leg fracture mechanics, and current bindings are only designed with release directions that cope with the forces involved in the latter of these instances. According to the research conducted by Johnson, Ettlinger, and Shealy over a period of 32 years in Vermont, ACL injuries are not related to the quality (the retention settings and release accuracy) of the release system of the binding [11]. This shows that current bindings are not truly designed to account for knee injuries. If they were, there would be a clear correlation between the quality of the release system and ACL injuries. Therefore, the bindings must be modified if they are to account for ACL injuries.
THE MECHANISMS OF AN ACL TEAR
Most injuries that affect the human body can be described in a biomechanical fashion. Understanding of knee injuries has especially benefitted from biomechanical studies—those that relate the physics of the motion of the human body to its biology. This understanding is critical if to correctly modify equipment to prevent specific loads on the knee's ligaments. Before describing the mechanics of the injuries, it is important to first define axes, forces, and moments in relation to the ski-boot-binding interface. These are set in Figure 4.
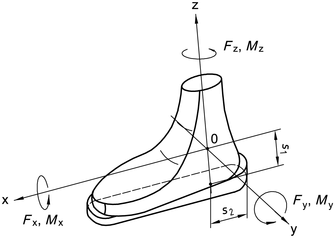
FIGURE 4 [1]. The binding axes as defined by The International Organization for Standardization (ISO)
In skiing, the two most common knee injury mechanisms are known as the phantom foot and the anterior drawer [13]. The phantom foot situation, also known as the backward twisting fall, usually occurs when a skier falls backward and catches an edge as he puts all his weight on the inside of the downhill ski, as in Figure 5. This very often occurs when the skier attempts to recover and stand back up in the midst of a backward fall. As the skier falls, his body twists in the downhill direction. At the point of injury, the hips are below the knees (which are flexed over 90 degrees) and the tail of the ski, in combination with the stiff back of the boot, creates an abnormal, unnatural twisting load on the tibia. This results in internal rotation of the tibia and valgus, blowing out the ACL [13]. Mechanically, this situation results directly from the fact that this mechanism applies a force on the inside of the edge of the ski directly on the z-axis of the tibia. Therefore, the binding does not register the force or release because there is no lever arm.
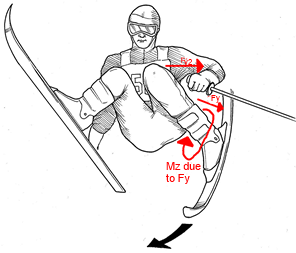
FIGURE 5 [12]. Skier in a typical phantom foot situation. Moment Mz due to Force Fy causes interior rotation of the tibia while Force Fy2 causes valgus of the joint. Along with knee flexion of greater than 90 degrees, these factors result in a torn ACL.
A boot induced anterior drawer situation usually occurs while a skier lands off balance to the back while jumping. As he or she lands, his or her legs straighten out instinctively, resulting in a landing on the tail of his or her ski. The ski, in combination with the stiff boot, causes a moment about the y-axis. Since most bindings have no way to sense this kind of force, the binding will not release, or will release too late. The force pushes the tibia forward in relation to the femur, which tears the ACL [13]. Figure 6 is a visual representation of this mechanism.
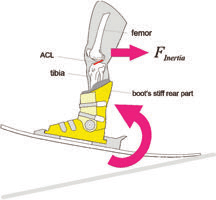
FIGURE 6 [1]. The force due to impact on the tail causes a moment, My, about the ski, pushing the boot and the tibia forward relative to the femur, tearing the ACL
BINDINGS OF THE FUTURE
The next step in the development of bindings is to take into account the injury mechanisms previously described. By doing so, skiing can become an even safer sport. By reducing injuries significantly, engineers can have a profound impact on skiing. This section describes how exactly engineers can mitigate ACL injuries in skiing, both in the present and in the future, concentrating particularly on the mechanics of the potential solutions.
Before a discussion about "safer" bindings, it is important to discuss what exactly makes bindings safe. There is a good reason for the stagnation of binding design in the last 20 years. The central aspect of this issue is premature release. This term refers to the inadvertent, undesired release of the binding mechanism during an activity that otherwise would not have been harmful. Such an occurrence can range in importance from an inconvenience that makes skiing less fun to a life threatening experience that can leave a skier's body traumatized for the rest of his or her life. Current binding standards, as already discussed, are designed to maximize the protection against tibia fractures. However, they also have a second, equally important function: minimize premature release. To this end, conventional bindings do a wonderful job in that most skiers will never have a premature release. Any suggested binding design must take into account this important factor. If a binding releases in situations when it is not intended to, it must immediately be reevaluated. Premature release poses the most challenging problem for any engineer attempting to create a new binding. Therefore, all of the suggested solutions to the ACL problem in this paper will also examine the safety of the binding in terms of premature release.
The Mechanical Solution
All bindings currently on the market can be classified as mechanical bindings. These release when a sufficiently large force acts on a proper area of the ski-boot-binding interface. However, as has been discussed, the forces due to the mechanisms associated with ACL injury do not trigger a binding release until it is too late, if at all. Therefore, a mechanical solution to this problem is the incorporation of additional degrees of freedom. Most conventional bindings have only two degrees of freedom for release: the moment about the z-axis due to a lateral force on the ski (Fy) and the moment about the y-axis due to a forward force (Fx). Adding even one more degree of freedom to counteract one of the ACL injury mechanisms could dramatically reduce injury risk.
Thought to be the most common injury mechanism in skiing, the phantom foot has received the majority of the focus when it comes to specialized bindings [12]. The idea behind preventing the phantom foot stems from the key problem of the injury: the idea that a force with a lever arm of zero produces no moment to trigger release. Therefore, a way to combat this problem is the addition of a switchable center of rotation (pivot point). Allowing the boot to pivot about the toe as well as the heel causes a movement of the triggering axis. This new triggering axis can sense the force that previously would have gone undetected [1]. Of any ACL injury reducing binding concept, this type has the largest market presence by far due to KneeBinding, with over 300 retail partners in six countries and 36 States [14] This small startup equips bindings with their trademarked PureLateral technology, which provides the dual pivot point described above [14].
Carl Ettlinger, one of the foremost authorities on safety in skiing, has designed and patented another type of binding based on his extensive research into the phantom foot injury. The design is primarily derived from a specific study that he conducted, which breaks the ski up into four quadrants in the x-y plane, which are shown in Figure 7 [15].
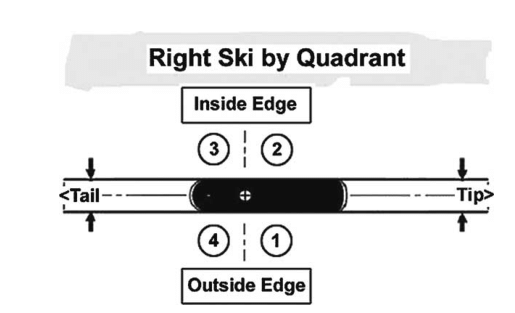
FIGURE 7 [15]. Quadrants as defines by Ettlinger and his team. The inside edge to the rear of the ski is of particular interest
The team found that current binding release settings provided adequate protection in three of the four quadrants without appreciable risk of unnecessary release. However, in the third quadrant, the inside edge toward the tail of the ski, the team found that conventional bindings' release settings were well above the recommended retention requirements developed by the authors in their experimental binding. This finding is displayed graphically in Figure 8 [15].
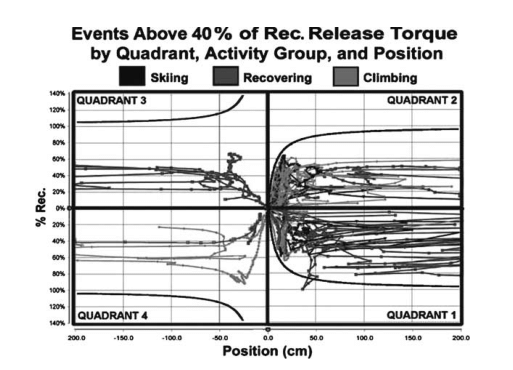
FIGURE 8 [15]. In Quadrant 3 (the ski's rear inside edge) events above 40% of the recommended release torque never approach the release requirement for a binding mechanism, represented by the asymptotic solid line.
They argue that the attenuation of binding settings by over 60% when a load is applied to the third quadrant would be an effective way to mitigate ACL injuries [15]. Keeping this observation in mind, Ettlinger designed his binding to have a reduced release requirement in the quadrant in question (referred to as the third quadrant in the study). The binding utilizes a pivoting system of platforms atop the ski. This system is configured so that only a force in the third quadrant can cause the platforms to pivot which allows for a secondary release system (or attenuated release system). Once the platforms pivot, the toe of the boot slides out of the toe piece much like in a conventional binding. To control the sensitivity of this release mechanism, Ettlinger uses sliding hold down mechanisms and a screw and spring system. These springs can be tightened or loosened to decrease or increase the likelihood of release. One can imagine during a phantom foot incident how the heel of the boot would turn toward the center of the ski, exerting a force on the third quadrant. This force could pivot the "trigger platform" under the binding counterclockwise relative to the ski. Once the trigger platform has pivoted, it opens the latch, allowing the secondary toe release platform to pivot clockwise [16]. Figure 9 shows the pivoting release described above.
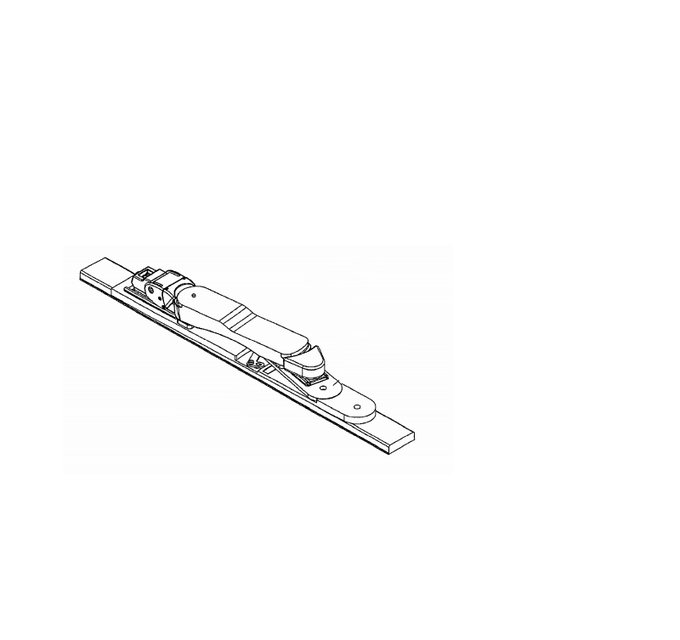
FIGURE 9 [16]. The plate system underneath the binding pivots in response to a strong enough force on the inside edge toward the tail of the ski. The boot freely slides out before a phantom foot can occur
As mentioned above, another mechanical solution that has been implemented is a vertical toe release mechanism (see Figure 2). When a large load is applied upwards in the toe piece, a binding of this mechanism will release upwards. In theory, this solution would directly counteract the anterior drawer mechanism. In this situation, much of the force exerted by the ski tail creates a vertical load on the toe piece and a vertical system will release. Many companies have tried to implement this technology, but currently, the market is almost devoid of them. The major drawback is premature release. An unwarranted release often can be much more dangerous than a torn ligament and it is a major problem for vertical release bindings. More advanced skiers (who tend to ski faster and on more challenging slopes with lots of bumps and moguls) find that this sort of binding will release due to ordinary loads during their harder runs. Therefore, they are inclined to crank up the DIN on the vertical release, essentially negating its positive effects towards the anterior drawer. However, for a beginner or moderate level skier, who does not plan on doing extreme runs, this type of binding can provide adequate protection from the boot induced-anterior drawer [1].
The solutions described in this section are good options for market implementation because of their sustainability; they improve consumer quality of life and increase the safety of skiers. Also, as KneeBinding has already proven, it is possible to manufacture a binding that protects ACLs and sell it for about the same price as any other binding. Ettlinger's design would also be reasonably priced. Although the pivoting plate system is reasonably complex, the parts required to make it are simple (screws, bolts, metal plates, etc.) and can be acquired inexpensively [16]. Due to the low parts and manufacturing cost associated with the design, the bindings would sell at a normal price on the market, and would represent another cheap, safe, and sustainable alternative to conventional bindings.
The Mechatronic Solution
The other major category of "safer" ski binding designs is mechatronic bindings. This category of binding makes use of electrical sensors in the boot, binding, and lower leg to assess whether or not the binding should release in a high-load situation. The primary goal of mechatronic bindings is to reduce the amounts of pre-releases during non-crash situations and increase the number of releases in critical load situations when release is actually needed. As a result, they allow for increased degrees of release freedom which ease forces on the knee in a critical load situation [17].
Neptune and Hull designed a prototype binding that addresses these issues and the issues with other current bindings discussed previously [18]. It features a system of pressure and force sensors that relay messages to electromechanical release mechanisms elsewhere in the binding. What makes the design unique to all other binding designs is that the toepiece, which is a modified Tyrolia 360D part, is stationary and offers no degrees of release freedom (the Tyrolia 360D originally offered a horizontal degree of release). Instead, it acts as a force measurement device. The toepiece contains a dynamometer in which the Tyrolia stock tension spring, used to measure the lateral force delivered by the boot, was originally located. This dynamometer can then send signals to the heelpiece of the binding, where the actual mechanical release function occurs. Additional sensors are located in the Anti-Friction Device (AFD) that measure forces involving the vertical release degree in the heel of the binding. Specifically, this part of the system assesses the forces related to the boot sole anterior bending moment, which covers all other degrees of freedom not addressed by the toepiece dynamometers. The AFD consists of beams with strain gauges on which the boot sole rests when it is engaged in the binding. The normal force of the boot sole on the strain gauges creates dynamometer signals that, like those from the toepiece, are relayed through the binding's circuitry to the mechanisms in the heelpiece [18].
The heelpiece is where all the mechanisms for releasing the boot are contained. As stated earlier, there are no release mechanisms in the toepiece, a feature unique to this particular binding. In this design, the heelpiece serves two functions: to release the binding when a threshold force by the boot is reached and to provide the system with elasticity to help prevent pre-release of the binding. The system allows for elasticity in three directions. There are two rotational degrees of elasticity that allow the boot to shift slightly in the binding without the binding "thinking" a release is necessary. This feature is key when skiing on rough, variable terrain because of probable constant forces on the binding from the boot that could be mistaken as critical load forces. The rotational elasticity helps to prevent inadvertent pre-release of the boot from the binding. Additionally, like most bindings, Neptune and Hull's binding also has a degree of elasticity longitudinally along the length of the boot which helps the binding to function properly even when the ski is under high load; in a steep, fast turn, for example. When the ski is flexed, the heelpiece is free to slide backwards on a spring-loaded rail, allowing consistent forward pressure to be applied from the toe of the boot to the contact point in the toepiece and from the heel of the boot to the contact point in the heelpiece. This is important because without consistent pressure at the boot-binding interface, the binding will not maintain the release characteristics that it is designed to possess and all release designs would not be effective [18].
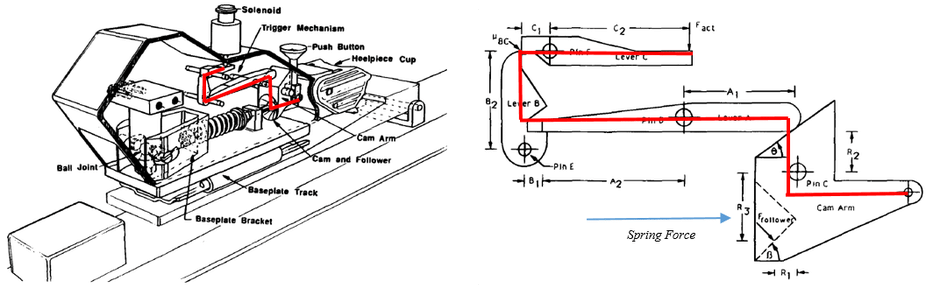
FIGURE 10 [18]. Diagrams showing the mechanism of the heelpiece in Neptune and Hull's prototype binding. The bottom diagram shows the highlighted lever portion, blown up for ease of viewing.
Aside from the circuitry utilized in the design, the actual release mechanism of Neptune and Hull's prototype binding is really rather simple. It consists of a series of levers in the housing of the heelpiece that connect the heel cup—the part that holds the boot in place and provides a contact point between the boot and binding—to a solenoid that actively controls the release function of the binding. These levers, shown from two different angles in Figure 10, create a mechanical advantage that allows for a relatively low-force power source to be used to release the boot from the binding by way of a loaded spring. In this case, the power source is a 27 Newton solenoid that is connected through the binding's circuitry to the dynamometers in the toepiece and AFD. When activated by the dynamometers, the solenoid provide the necessary force to move Lever C out of the path of Lever B in the Figure 10. This, in turn, releases the rest of the system, including the heel cup, from its resting, high-sprung state and releases the stored potential energy from the spring. It is this energy form the spring that provides the high force necessary to physically cause the binding to release [18].
The biggest benefit of this type of binding is that, since it has a more highly advanced release mechanism than conventional bindings, settings can be finely tuned to release exactly when necessary [17]. The forces to the knee involved in the phantom foot and the anterior drawer situations can be safely dealt with without sacrificing any of the binding's ability to still prevent tibia and other lower leg fractures. While Neptune and Hull's design is still only a prototype, mechatronic bindings definitely have a place in the safe binding market in the near future. Their ability to account for the forces involved in almost all leg injuries in skiing is unmatched by any other category of binding currently being discussed.
However, as of today there is one major flaw with the incorporation of mechatronics into ski bindings. The issue at hand is that these bindings are incredibly expensive to manufacture due to the complex electronic circuits and sensors that they employ [1]. Therefore, mechatronic bindings are currently unsustainable since they are too expensive for anyone to buy. This cost opposes the sustainable improvement in quality of life and safety that comes with such an advanced binding. The goal for the future of mechatronic bindings should be to reduce cost enough to make them truly sustainable by reducing the cost of manufacturing, the price, and keeping the same improvements of function.
A SUSTAINABLE MENTALITY
As an idea, sustainability is multifaceted. One could say that sustainability means cost effectiveness, eco-friendliness, or easy to manufacture. However, it can also include the idea of improved quality of life. In this case, quality of life can be defined as peace of mind, safety, and health. By implementing bindings that reduce ACL injuries, the overall quality of life of skiers will improve. Safer bindings will give skiers greater peace of mind, and decrease injuries in the population.
Another sustainable aspect of knee injury reducing bindings is that they are, in fact, very cost effective, when the bigger picture is considered. ACL injuries are extremely expensive affairs. The average cost of an ACL injury is about $35,000 [19]. However, that is just the average. For many, depending on the severity of the injury, the procedure can cost as much as twice that figure [20]. Fortunately, people with medical insurance do not have to pay that amount out of pocket; they will only have to pay a fraction of the total cost. Still, the huge costs exist as a strain on the economy. Somewhere along the line, someone will have to pay for the injury. For hospitals, much of the cost associated with patients with ACL injuries is efficiency. In areas where skiing is highly popular, such as Boulder, Colorado, Salt Lake City, Utah, and Portland Oregon, a high amount of emergency room traffic is due to skiers and their injuries. Non-life-threatening injuries such as ACL tears can cause backups that prevent patients with more serious ailments from receiving prompt care. By reducing ACL injuries, the bindings discussed can save a lot of money, time, and resources of hospitals and those that suffer the injury [21].
CONTINUING DEVELOPMENT
Creating safer bindings is an endeavor that a small group of researchers in the skiing industry have been working towards for decades. Many obstacles prevent the widespread adoption of ACL-friendly bindings in the market. Although engineers possess extensive knowledge of the biomechanics of the injury, developing a mechanical piece of equipment that reacts to very specific forces is extremely difficult, and incorporating mechatronics, while interesting, will not be economically viable for a long time.
Perhaps the central aspect of the problem is the skiing industry's traditional standards. The lack of progression of skiing equipment to protect knee injuries is evidence that companies are unwilling to go outside of the box and risk uncharted territory outside the types of release governed by the standards. The research that led to this paper has revealed a glaring deficiency on the market of innovative bindings. The skiing safety community has been aware of ACL injury mechanics like the phantom foot for over 20 years. However, over that time, bindings have undergone very little evolution. Years of research have been devoted to ACL injuries in skiing. Manufacturers and engineers have all the necessary information and technology needed to develop better bindings. In the future, in order to cultivate innovation in the binding market, new standards must be established, using the biomechanics of the injuries. After a set of concrete standards are defined, the industry will more readily implement the technology. Therefore, further research into this subject matter should not only examine the ways that ski bindings can be improved to mitigate ACL injuries, but also how standards can be used to regulate whatever technology the research focuses on. Armed with a brilliant new design and a set of irrefutable standards, binding companies could revolutionize skiing safety and reduce one of the most undesirable sports injuries in the world.
REFERENCES
[1] V. Senner, F. I. Michel. S. Lehner. (2013). "Ski Equipment-Related Measures to Reduce Knee Injuries." International Sports Engineering Association. (Online article). http://www.sportsengineering.org/wp-content/uploads/2013/1 2/ISSS-broschure_bfu-TUM.pdf.
[2] "The Health Benefits of Sport and Physical Activity." Sport and Development. (2013). (Online Article). http://www.sportanddev.org/en/learnmore/sport_and_health/the_health_benefits_of_sport_and_physical_activity/
[3] R. Johnson, C. Ettlinger, J. Shealy. (2008). "Update on Injury Trends in Alpine Skiing." Journal of ASTM International. (Online article). http://www.astm.org/digital_library/journals/jai/pages/jai102046.htm.
[4] C. F. Finch, H. L. Kelsall. (1998). "The Effectiveness of Ski Bindings and Their Professional Adjustment for Preventing Alpine Skiing Injuries." Sports Medicine. (Online article). http://www.ncbi.nlm.nih.gov/pubmed/9680660.
[5] "Anterior Cruciate Ligament Injuries (Isolated)." University of Minnesota Orthepedics. (2012). (Website). http://www.sportsdoc.umn.edu/Patients_Folder/Knee/aclreconpat/aclreconpat2.htm.
[6] B. Pennington. (2014). "Lindsey Vonn's Injured Knee Will Keep Her Out of Sochi Games." The New York Times. (Online article). http://www.nytimes.com/2014/01/08/sports /olympics/lindsey-vonn-wont-ski-at-the-sochi-games.html.
[7] S. Masia. (2012). "Release! History of Ski Safety Bindings." International Skiing History Association. (Online Blog Post). http://skiinghistory.org/history/release-history-safety-bindings.
[8] "Ski Bindings." Mechanics of Sport. (2012). (Online article). http://www.mechanicsofsport.com/skiing/equipment
/bindings.html.
[9] "Rossignol Axial2 120 Ski Binding." Evo. (2013). (Website).http://www.evo.com/shop/ski/bindings.aspx.
[10] "DIN Setting Calculator." Dinsetting.com. (2011). (Website). http://www.dinsetting.com/index.htm.
[11] C. F. Ettlinger, R. J. Johnson, J. Shealy. (2006). "Functional and Release Characteristics of Alpine Ski Equipment." Journal of ASTM International. (Online article). http://enterprise.astm.org/subscription/digital_library/stp/pages/stp39659s.htm.
[12] C. F. Ettlinger, R. J. Johnson, J. Shealy. (1995). "A Method to Help Reduce the Risk of Serious Knee Sprains Incurred in Alpine Skiing." The American Journal of Sports Medicine. (Online Article). http://www.ncbi.nlm.nih.gov/pubmed/8526266
[13] S. Freudiger, N. E. Friederich. (2000). "Critical Load Cases for Knee Ligaments at Skiing—An Engineering Approach." Skiing Trauma and Safety: Thirteenth Volume. (Online article). http://enterprise.astm.org/subscription/digital_library/stp/pages/stp12874s.htm.
[14] "Technical and Background Information." Knee Binding. (2012). (Online write-up). http://www.kneebinding.com/KB-InformationCenter3.aspx.
[15] C. F. Ettlinger, D. Dodge, R. J. Johnson, J. E. Shealy, M. Sargent. (2010). "Retention Requirements for Alpine Ski Bindings." JAI. (Online article). http://enterprise.astm.org/subscription/digital_library/journals/jai/pages/jai102978.htm.
[16] C. F. Ettlinger, D. J. Dodge. (2009). "Alpine Ski Binding Having Release Logic For Inhibiting Anterior Cruciate Ligament Injury." (Patent). Patent No.: US 7,762,572 B2.
[17] M. L. Hull. (1985). "A Survey of Actively Controlled (Electronic) Snow Ski Bindings." Skiing Trauma and Safety: Fifth International Symposium. (Online article).
[18] R. R. Neptune, M. L. Hull. (1996). "Electromechanical Ski Binding with Release Sensitivity to Torsion and Bending Moments Transmitted by the Leg." Skiing Trauma and Safety: Tenth Volume. (Online article). http://enterprise.astm.org/subscription/digital_library/stp/pages/stp37942s.htm.
[19] T. L. Chancellor. (2011). What is the average cost of ACL Surgery? eHow. (Online article). http://www.ehow.com/about_5398546_average-cost-acl-surgery.html
[20] C. French-Owen. (2013). The Cost of an ACL Injury. Calv.info. (Online Blog). http://calv.info/the-cost-of-an-acl/
[21] R. C. Mather III, L. Koenig, M. S. Kocher, et al. (2013). The Journal of Bone and Joint Surgery. (Online article). http://jbjs.org/data/Journals/JBJS/927628/1751.pdf
ADDITIONAL SOURCES
"ACL Injuries From Alpine Skiing." California Pacific Orthopaedics and Sports Medicine. (2013). (Online article). http://www.cposm.com/sports-medicine/skiing-injuries/acl-injuries.
R. Bahr, T. Krosshaug. (2009). "Understanding Injury Mechanisms: A Key Component of Preventing Injuries in Sport." British Journal of Sports Medicine. (Online article). http://www.ncbi.nlm.nih.gov/pubmed/15911600.
B. Caldwell, D. Landry, M. L. Hull. (1993). "A Mechanical Ski Binding with Heel Release Activated by the Bending Moment at the Boot Sole." Skiing Trauma and Safety: Ninth International Symposium. (Online article). http://enterprise.astm.org/subscription/digital_library/stp/pages/stp25570s.htm.
http://enterprise.astm.org/subscription/digital_library/stp/pages/stp46642s.htm.
S. Freudiger, W. Vogt, D. Wirz. (2010). "Relative Motion of ACL Insertion Points In Vivo: A Case Study, Including Skiing Maneuvers." Journal of ASTM International. (Online article). http://www.astm.org/digital_library/journals/jai/ pages /jai102831.htm.
R. Johnson, C. Ettlinger, J. Shealy. (2003). "What Do We Know About Ski Injury Research that Relates Binding Function to Knee and Lower Leg Injuries?" Skiing Trauma and Safety: Fourteenth Volume. (Online article). http://enterprise.astm.org/subscription/digital_library/stp/pages/stp10960s.htm.
U. Lindsjö, H. Ekström, J. Gustavsson. (2010). "Release and Retention in Apline Ski Bindings." International Journal of Sports Medicine. (Online article). http://www.ncbi.nlm.nih.gov/pubmed/6874175.
V. Senner, F. I. Michel, S. Lehner, O. Bru¨gger. (2013). "Technical Possibilities for Optimising the Ski-Binding-Boot Functional Unit to Reduce Knee Injuries in Recreational Alpine Skiing." International Sports Engineering Association. (Online article). http://link.springer.com/article/10.1007% 2Fs12283-013-0138-7#page-1.
J. E. Shealy, I. C. F. Ettlinger, R. J. Johnson. (2005). "Using Signal Detection Theory as a Model to Evaluate Release/Retention Criteria in Alpine Skiing." Journal of ASTM International. (Online article). http://www.astm.org.
G. S. Wunderly, M. L. Hull. (1989). "A Biomechanical Approach to Alpine Ski Binding Design." International Journal of Sport Biomechanics. (Online article). http://journals.humankinetics.com/jab-back-issues/jabvolume 5issue3august/abiomechanicalapproachtoalpineskibindingdesign.
Comments